Will protein design tools solve the snake antivenom shortage?
4k words, 19 minutes reading time
(Note: In case you were at ICLR and missed this, I did an interview with Sergey Ovchinnikov (of protein design fame) last week!)
Introduction
There has been a fair bit of discussion over this recent ‘creating binders against snake venom protein’ paper from the Baker Lab that came out earlier this year, including this article from Derek Lowe.
For a quick recap of the paper: the authors use RFDiffusion (a computational tool for generating proteins from scratch) to design proteins that bind to neurotoxic protein found in snake venom, preventing it from interacting with the body. They offer structural characterization results to show binding between their created protein binder and the protein in question (three-finger toxins), and in-vivo results in mice demonstrating that their created protein confers protection against the designed protein as proof.
It’s excellent work, and potentially one of the first times that RFDiffusion has been used in such a straightforward way for a clearly useful problem (though others disagree with me on this being the first time). But, there’s a few obvious questions here: why computationally design binders against venoms if we already have antivenoms available on the market? What further work remains to be done to create binders against any arbitrary venom? And when can we expect that?
I asked these questions, fell down a surprisingly rabbit hole, and decided to compile the results together to release as an article. This is the result.
Let’s get started!
The dismal state of antivenom production
Vaccines are the prototypical ‘clearly good for humanity, but terrible profit incentives’ drug. You spend billions on R&D and clinical trials, give someone a shot once, and then they’ll never need the drug again (or need it once every decade). As such, you end up with these insane cases of promising vaccines being left by the wayside for decades due to economic reasons (see the malaria vaccine debacle), with millions of lives being lost in the process.
In many ways, antivenom production mirrors the problem of vaccines, but on a smaller scale.
But you needn’t take my word for it! As it happens, the Works in Progress magazine has already published a lengthy essay on how horrifically bad the state of antivenom manufacturing is. As such, instead of taxing my own brain, I will rely on quotes from their very well written piece to cover the main points.
On how many people are affected by snake bites:
Venomous snakebites kill between 81,000 and 138,000 people each year, and leave another 400,000 with permanent disabilities. This ranks it among the deadliest of neglected tropical diseases, alongside better-known ailments such as typhus and cholera.
On the cost of antivenoms:
The average cost of antivenom alone in sub-Saharan Africa was $124 in 2010, a steep price to pay for the 40 percent of sub-Saharan Africans who live on less than $1.90 per day. And that doesn’t even include the cost of the care or facilities. A survey of 129 Bangladeshi snakebite victims found that over 40 percent had to take out loans to meet the costs.
On the state of the antivenom shortage:
Need for antivenom is increasing each year, yet the supply of quality antivenom fails to keep up. A 2011 study found six companies producing antivenom sold in sub-Saharan Africa. These companies produced 410,500 ampoules of antivenom, just enough to treat 96,000 cases. That’s only one third of the total estimated cases on the continent….The global antivenom market in 2016 was valued at $1.1 billion and was projected to reach $1.5 billion by 2021. It instead fell to $1.02 Billion.
Why there is a shortage at all:
The mess of poorly tested, mislabeled, and at times outright fraudulent products has resulted in the current predicament: a market for lemons. Confidence in antivenom treatment has fallen…..Even if antivenom production at high throughputs and low prices would be profitable over a longer time horizon, producers have been unwilling to make the large up-front investments necessary to achieve that scale, in no small part due to uncertainty over whether governments and clinics would follow through on promises to buy at those prices. Governments and clinics, on the other hand, have become wary of making large purchase orders after being burned one too many times on promises of cheap antivenom.
So, with the thesis of why we’d want better antivenom cleared out, we can continue. Before we start discussing how antivenom may be improved, we should first understand venom.
A primer on snake venom heterogeneity
Keep in mind, snake venom is not just one thing, but rather an umbrella term for a cocktail of proteins (and small molecules, though these are less important). The therapeutic issue here is that the composition of the toxin can vary at four increasing levels of taxonomic specificity:
Between snake families (obvious)
Between snake species (obvious)
Between snakes of the same species (less obvious)
Within the same snake over time (perplexing)
Let’s go in order.
At the familial level, venom composition heterogeneity is the strongest. There are around twenty snake families in total, but only four are known to be venomous: Viperidae (vipers), Elapidae (elapids), Atractaspididae, and Colubridae. Of these, the latter two are typically left out in discussions of snake bites, as they are either quite shy, have relatively innocuous venom, or physically are unable to bite humans due to the location of their fangs (e.g. rear-fanged snakes). Because of this, we’ll focus on vipers and elapids. These two possess the strongest differences in venom composition.
Elapids venoms contain three-finger toxins (3FTxs, which is the one discussed in the Baker Lab paper), Phospholipase A2 enzymes, and Kunitz-type serine protease inhibitors. These toxins are primarily neurotoxic.
Viper venoms are predominantly composed of enzymatic proteins such as snake venom metalloproteinases (SVMPs), snake venom serine proteases (SVSPs), and L-amino acid oxidases (LAAOs). These enzymes primarily cause tissue destruction, hemorrhage, and coagulopathy.
So, obviously, entirely different classes of proteins, thus entirely different needed therapeutics. Simple, let’s keep two types of antivenom, one for each family.
But, while the broader strokes of proteins are different between snake families, there continue to be major deviations amongst species of the same family; the primary example being toxin compositions that are entirely unique to singular species. Naja nigricollis (Black-necked spitting cobra) venom uniquely contains cytotoxins in addition to neurotoxins, though, admittedly, the cytotoxins themselves aren’t especially dangerous unless injected near the eyes. On a more fatal note, Dendroaspis polylepis (Black mamba) venom has an especially high concentration of dendrotoxins, which are neurotoxic enough to cause death in an hour after a bite. But there also exist cases of extraordinary compositional heterogeneity amongst snake species venoms; no unique proteins, but unique clinical impacts. The prototypical case here is Daboia russelii (Russell’s viper), which uniquely produces a highly procoagulant venom despite lacking wholly unique proteins, leading to paradoxical blood clotting, kidney failure, and eventually death.
Okay. Two antivenoms for every snake bite was a pipe dream, perhaps we’ll need to scale things up. Perhaps an antivenom for every venomous snake species? There’s only 600~ of them. That’s not so bad.
But, once again, there’s another level of heterogeneity. Snake venom composition can vary not only amongst species, but within a species too. Famously, the Crotalus scutulatus (Mojave rattlesnake) has populations that produce completely different types of venom. Some populations produce venom with presynaptic neurotoxins (type A), while others lack it entirely and instead produce venom rich in hemorrhagic metalloproteinases and serine proteinases (type B), — both of which require completely different antivenoms. And, sometimes, there are snakes that possess both types of these venoms. Most worrying of all is that the geographic variation of all of these venom types is decently intermixed; sometimes separated by cities but sometimes separated by only a few miles. A nearly identical phenomenon is noted in Crotalus horridus (Timber rattlesnake).
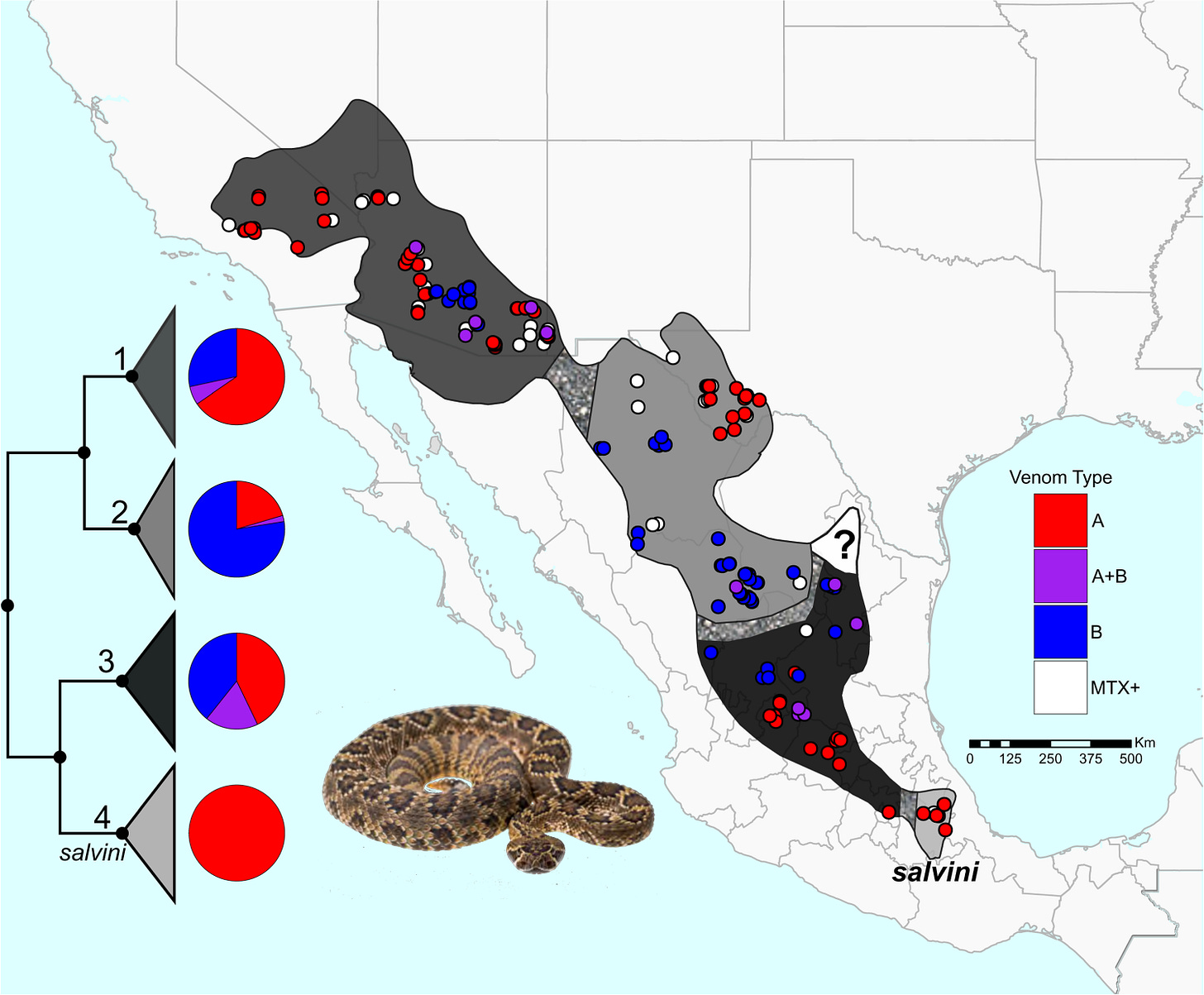
Okay. Okay okay okay. We'll need different antivenoms for different populations of the same species. This does appear to be an edge case…so maybe it doesn’t bump up the number of antivenoms we need to keep in stock much. Is that it?
One last bit. Even species + region specificity isn't enough, because venom composition can vary within a single snake over its lifetime.
A dramatic example of this is in the family Pseudonaj (Australian brown snakes), which have non-coagulopathic venom as juveniles, but switch to coagulopathic venom as adults. Other than for one specific species, which continue to have non-coagulopathic venom as adults! Why does this occur? Often referred to as venom ontogenetic shift, it is likely driven diet-based selection pressures. And these venom shifts aren’t sudden snap changes in proteomic venom composition of their venom as they age, but rather a gradual shift over time. Because of this, there will be no ‘young snake’ antivenom and ‘old snake’ antivenom, since it’s all a spectrum.
Luckily, that’s the last layer of venom complexity.
Given all this, one may start to view venom as an almost...cancer-y phenomenon. It's all so heterogeneous, in the sense that what works for one case may be completely ineffective for another, even when they appear superficially similar. Even the theoretical framework for why such venom differences even exist is fuzzy. Like, we can point to diet-based selection pressures for ontogenetic shifts, but even that doesn't fully explain the geographic variation we see in species like the Mojave rattlesnake we mentioned earlier.
But unlike cancer we, at least theoretically, have a real solution for it: anti-venom. Antivenom that, admittedly, must account for the venom heterogeneity specific to the snake type, species, region, and age of the snake. But a solution nonetheless. Speaking of, that’s our next topic.
But, before we move on, it’s worth noting that this section has primarily dealt with the proteomic composition of venom. What of the small molecules in them? There is unfortunately relatively little information on them. We know they exist, one review paper specifically says ‘salts, amino acids, hormones, nucleosides, neurotransmitters, and polyamines’ have been found in venoms. But it is, from literature review alone, unclear what exactly these do. Given that off-the-shelf antivenoms seem to largely ignore the existence of these, I’m assuming the small molecules are largely there to amplify or stabilize the proteomic elements of the venom. As such, I too will ignore them in the following section.
A primer on snake antivenom
At a base level, antivenoms are relatively simple. As with nearly everything [bad] floating around in our body, the best way to deal with venoms is to simply prevent it from interacting it with anything. In the case of viruses, bacteria, and even cancer, one way the body goes about doing that is via antibodies (something I’ve written at length about). To create an antivenom involves doing the exact same thing. Simply produce en-masse antibodies that bind to the proteins that the snake bit you with, preventing it from interacting with anything else in your body, and you’re cured!
This may raise an immediate question: is the antivenom produced by one snake only applicable to that exact species? How much of an issue is the venom heterogeneity problem we’ve spent so much time talking about?
Well...it’s complicated. In the absolute ideal case, if you want to ensure that no case of snake envenomation goes untreated, you need to have snakes species that matches every single possible venom composition (the count of which is understudied) and are willing to consistently produce venom (a notorious problem with some snakes, such as coral snakes). Due to this, most antivenoms have, almost by necessity, become hyperspecialized. One review paper has this insane statement: For example, India has more than 60 venomous species of snake, and there is no specific antivenom against most of them. If you are bitten by a snake that is not one of the ‘big four’ (spectacled cobra, common krait, saw-scaled viper and Russell’s viper), the antivenom will be largely ineffective.
So how many venoms do we need to produce an effective antivenom against them? Well, while I did say there are 600 species of venomous snakes worldwide, only 200 produce venom that’d be dangerous to a human. But remember, we have the complexity of regional and ontogenetic differences to deal with. And, unfortunately, it is extremely unclear from the literature how many extra antivenoms that these two extra layers of complexity would demand.
But perhaps we’re going down the wrong route entirely. Instead of trying to come up with a universal list of all possible venom compositions, we could perhaps reframe the problem. Instead of caring about the universe of venoms, we should care about the universe of proteins contained within all possible venoms.
Clinically speaking, this means moving away from thinking about monovalent antivenoms — antivenoms derived from a single species — and instead relying on polyvalent antivenoms — multiple different antivenoms that have been combined together. This way, we needn’t need keep 200 or so different vials of antivenom together, but group them together into a few dozen combinations. If you’re bitten by a snake in Australia (and don’t die in the next 15 minutes), we needn’t try to identify the exact species that bit you and provide that specific antivenom. Instead, you’ll simply be given the antivenom derived from all snakes that live in Australia. Or at least, at least some of the major snake species, with the assumption that there will be sufficient cross-neutralization (also called (paraspecific neutralization) for snake venom that we lack.
And, practically speaking, this is exactly what is done. At least in Australia, where there is a polyvalent antivenom licensed to treat envenomation by any of the major Australian snakes (taipans, brown snakes, tiger snakes, death adders, black snakes, many of whom share toxic proteins). Of course, there isn’t a free lunch here; polyvalent antivenoms must be administrated at relatively heavy doses, since they are diluted across multiple venom targets. This means more antibodies floating around, increasing the likelihood of immune reactions such as serum sickness or anaphylaxis. The more complex the mix, the greater the risk. Additionally, because you aren’t actually covering all snake species, neutralization efficiency for at least some snake species might be nonexistent.
But let’s ignore the nuances here and assume a few sets of polyvalent venom are all we need, which dramatically simplifies the extreme levels of toxicity heterogeneity we just discussed.
How exactly do we get those antibodies? Especially given the extreme heterogeneity we’ve seen in snake venom? Once again, I’ve been somewhat scooped here by another (very recent) Works in Progress essay, who has this to say about the traditional process of producing antivenoms:
In the case of molecular complexity, consider antivenoms. The method to make antivenom has not changed much over the last 100 years. Venom is first milked from snakes and then injected into horses or sheep, which then produce multiple different antibodies and antibody fragments that bind to the venom….Blood is collected from these animals, the antibodies are purified from the plasma, and they are then given to people bitten by venomous snakes.
Why horse and sheep for antibody production, and not E. coli or yeast or mammalian cells? Mostly size. Horses and sheep are big animals that can produce large volumes of blood (and thus antibodies) compared to microbial systems, which are often more expensive than they are worth given the terrible economics of antivenoms. Plus, their immune systems are reasonably similar to ours, which means the antibodies they produce are more likely to work in humans without causing severe immune reactions.
But could we produce antivenoms through microbial systems? This would be a necessity if we wanted arbitrarily designed antibodies, instead of whatever an animal naturally produces in response to an injected venom. We’ll discuss this a bit more in the next section.
Sure! Plenty of startups have spun up to do it. The basic pitch of it is to simply go through the usual horse/sheep production process, find the antibodies that are made in response to the venoms, and get an E. coli cell to produce the same thing via genetic engineering. And so we got companies like Venomyx, founded in 2015, and VenomAb, founded in 2016.
How are they doing? Well…both are out of business. It is unclear why Venomyx shut down, but for VenomAb the reason for the shuttering was, as could be guessed, that delivering a return to investors would be nearly impossible. No technological infeasibility, just hard to make money off of.
At this point, you should understand three things:
Why there is an antivenom shortage
The heterogeneity of venoms
The associated heterogeneity + production difficulties of antivenoms
Armed with this context, we can finally answer the question: will protein design models help with the antivenom shortage problem?
Do computationally designed antivenoms actually solve anything?
In the two articles discussing the original Baker lab paper, both touch on how this work alone doesn’t solve the problem of snake venom. Why?, you may ask, don’t the in-vivo results show that they do?. Well, importantly, the in-vivo results aren’t actually designed protein versus snake venom, it is designed protein versus three-finger toxin. Derek Lowe specifically says:
These are still not going to snakebite cures by themselves, though, it has to be noted. There are other classes of nasty ingredients in snake venoms, with phospholipase enzymes near the top of the list.
But, really, that’s a minor point. Honestly, we’re nearing a future of having binders-on-demand for any arbitrary target through computational methods. Some would say we are already there, so having to deal with a few extra enzymes shouldn’t be a big issue.
Moreover, for how much we’ve gabbed about venom heterogeneity, we’ve also discussed that it may very well be that the whole problem doesn’t matter, and cross-reactive/polyvalent antivenoms save the day. Australian antivenoms were one case of this, but it could be taken way, way further. Just in 2024, researchers screened fifty billion antibodies to find one that bound to all three-finger toxins found in elapids, many of which are structurally quite diverse. Will we be able to find similarly universal antibodies for the metalloproteinases in viper venom? For the phospholipases? And even if we do, can we get all of them to work together in a single cocktail that neutralizes all venom types without introducing new safety issues?
Honestly, probably. It feels like a decent bet that similar ‘universalities’ will be found across the toxin family — there is necessarily not a ton of evolutionary divergence amongst venoms.
In time, methods like this may lead to a singular, extremely cross-reactive antivenom. One that contains only one, two, or a similarly small number of unique antibodies, but still manage to simultaneously neutralize the venoms of differing classes, species, regions, and ages of snake. No need to identify a snake to administer the correct antivenom anymore — if you manage to survive the trip to the hospital, there will be a cure waiting for you. We may very well live in the universe where these sorts of ‘holy grail’ antivenoms never actually comes to fruition, but I feel like theres decent enough reason to believe it is possible, so I will presume their existence for the rest of this essay.
So, am I saying that computationally designed antivenoms will herald the end of the antivenom shortage? Well, let’s say we have this theoretical universal antivenom, granted to us by our protein design overlords. Or maybe through phage display. Or maybe phage display combined with protein design methods to further optimize them, whatever.
Here’s one pessimistic future: remember, antivenoms remain an awful market to work in, even independent of how hard they are to make. At least vaccines have the economic benefit of first-world children needing them, often supported by wealthy governments or global initiatives providing guaranteed demand and thus ensuring profitability. Antivenoms lack this advantage: they predominantly affect low-income populations in developing countries, regions with limited purchasing power, inconsistent healthcare infrastructure, and unpredictable demand. No wealthy parent or government is routinely lining up to buy antivenom ahead of time, leading to the economic catch-22 we've already outlined.
It…doesn’t feel like this changes dramatically even if we have a perfect universal antivenom. The market does expand a bit, people may be more willing to trust and seek hospital treatments, and governments may have a clearer incentive to maintain consistent supplies. But fundamentally, one is still serving populations with very limited purchasing power and unpredictable demand. A perfect computationally-designed antivenom doesn’t guarantee market incentives. It simply makes a previously terrible market slightly less terrible.
In this world, protein design models change little about the status quo. At best, the thermostable properties of our hallucinated antivenoms — a phenomenon seen in both the Baker lab paper and also a weirdly consistent characteristic of many AI-generated proteins — makes the cold supply chain less of a bottleneck. That’s not nothing! But it may not be enough.
Yet…there’s also a more optimistic side, one that the first WiP essay we discussed alluded to. Quoting from there:
If antivenom producers can be made confident that large investments will pay off, they are more than happy to make them. If governments are confident the antivenom they receive actually works, they are more inclined to buy it. When people start returning from the hospital healthy, happy, and with their wallets intact they are likely to go again and recommend their loved ones do too. In the Bangladeshian survey, most victims and their relatives expressed they would be willing to discontinue traditional healing practices if hospital treatments were easily available.
If we’re to trust the Bangladeshian survey, universal antivenoms, stemming from protein design models, may be a panacea to the whole economic problem faced by antivenoms. Even if antivenoms are a bad market, a sufficiently good product may be enough to conquer the major issues.
But, remember, universal antivenom could only be produced via cellular production methods. Why? Because there is, as far as I can tell, no known methods to easily elicit arbitrary antibodies (as in, the universal ones we want) from animals, you need to genetically modify cells to do it. And non-animal antivenom production startups haven’t been particularly successful!
But perhaps history won’t repeat. The improved market opportunity may very well push pharmaceutical companies to reconsider cell-based production methods, since a trustworthy antivenom would skyrocket demand. And without the burden of animal management, venom procurement, and purification processes, this would push production costs down even more, making investment here further attractive.
In such a world, the antivenom shortage problem would be solved outright, becoming a very tolerable expense for anyone unlucky enough to be bit by a deadly snake. No antivenom company will earn tens of billions working here, but will likely comfortably recoup their investments and achieve modest returns. A sustainable market attractive to both public and private investors. And likely also one that is trusted as an area for non-profits/governments to sponsor.
Which world do we live in?
I’m unsure.
It feels like protein design models really only solve the first bit, the creation of near-universal antivenoms. While intellectually interesting, the utility of these antivenoms would rely on cell-based production of those antivenoms, which is a tall order. Not because it’s a hard problem; in many ways, it’s likely solved, given that we have mass-produced antibody drugs available on the market produced in cell-based settings. But, rather, because it requires a huge amount of upfront capital and engineering work.
And antivenoms don’t seem to historically be a place where many people are willing to make that investment. But, given a sufficiently good protein design model that produces a good-enough universal antivenom, maybe that’ll change. Who knows?
Not just horses apparently … https://www.nytimes.com/2025/05/02/health/snakes-universal-antivenom-tim-friede.html?unlocked_article_code=1.EE8.b1BN.gZ0RPnzYADAr&smid=url-share
A family member of mine got bit here in Australia and they didn’t even give them any anti venom as the risks of side effects wasn’t worth it. They said it was hell sweating it out so I would think there would even be a market here if you could make them more targeted!